The Effect of Sinecatechins on HPV-Induced Cell Growth and Apoptosis Induction
Stephen K. Tyring , MD, PhD, MBA
YAuthor Information, Copyright and License Information, Disclaimer of Liability
Abstract
Background: Green tea catechins possess a wide range of pharmacological properties, including antiviral, anti-infective, and immune-stimulating effects. Additionally, they have shown inhibitory effects on various enzymatic and metabolic pathways involved in cancer development. Catechins have been demonstrated to have preventive properties against proliferation in various cell lines and may have a direct virucidal effect. The United States Food and Drug Administration has approved a topical ointment formulation of sinecatechins derived from green tea catechins and other tea components for the treatment of external genital and perianal warts. The exact mechanism of action of sinecatechins in the elimination of external genital and perianal warts caused by human papillomavirus is not known but could be related to one or more of the mentioned mechanisms.
Objective: This study was conducted to investigate the potential of sinecatechins to inhibit growth in human cervical carcinoma cell lines infected with human papillomavirus.
Methods: The viability of tumor cell lines (CaSki and SiHa infected with human papillomavirus-16; HeLa and C4-I infected with human papillomavirus-18) was investigated as a parameter in a short-term viability assay (48 hours). This was followed by a long-term clonogenic assay (12-23 days) to determine the cytotoxic potential of sinecatechins as a parameter for cell viability and proliferation. This assay determined whether the observed effect in the viability assay was due to a delay in cell proliferation or a decrease in total cell count leading to cell death.
Results: According to the collected data, sinecatechins inhibited cell growth by 50% (GI50) in all four tumor cell lines at concentrations ranging from 160 to 360 uM. C4-I cells were the most sensitive to treatment with sinecatechins, with a lower GI50 (~34uM). The total GI was obtained at 625uM sinecatechins (40uM for C4-I) in a 48-hour assay and growth inhibitory potential detectable one hour later. Clonogenic analyses confirmed the cytotoxic potential of sinecatechins with a concentration-dependent decrease in colony numbers. While sinecatechins significantly reduced the number of surviving HeLa cells at a concentration of 200uM, surviving SiHa cells were almost completely eradicated at a concentration of 600uM.
Solution: Sinecatechins exhibited growth inhibitory potential in all four human papillomavirus-infected tumor cell lines, which could be attributed to the induction of apoptosis mediated by cell cycle deregulation. Additionally, this antiproliferative effect may contribute to the overall cancer-preventive function of sinecatechins and their potential direct antiviral activity, which could contribute to the clearance of external genital and perianal warts.
The medicinal properties of tea leaves have been known for thousands of years. Tea leaves produced from the Camellia sinensis plant contain two unique bioactive compounds: flavonoids and methylxanthines. Methylxanthines, including caffeine, theobromine, and theophylline, are a group of alkaloids found in small amounts. Flavonoids, a subtype of which are known as catechins, are polyphenolic compounds that constitute 25 to 35 percent of green tea and are responsible for many of its beneficial effects. Epigallocatechin-3-gallate (EGCg), a significant component of catechins, is considered the dominant therapeutic agent derived from green tea. Catechins, including EGCg, have a wide range of beneficial effects, including helping to prevent chronic diseases ranging from cancer to cardiovascular diseases.
Biological and pharmacological spectrum of catechins. The spectrum of biological and pharmacological properties of green tea catechins includes anti-angiogenic activity, anti-inflammatory and immune-stimulating activity, and antimicrobial potential.
The antineoplastic activity of EGCg involves affecting tumor cell growth by inhibiting proliferation and inducing apoptosis. EGCg has been shown to inhibit growth and induce apoptosis in head and neck squamous cell carcinoma, liver, ovarian, prostate, breast, and cervical cell lines. Its antiproliferative and anticarcinogenic effects have also been demonstrated in vitro in lung cancer cells, colon tumor cells, as well as lymphoma and leukemia cells. Tumor reduction induced by EGCg has been demonstrated in breast cancer cell lines. EGCg has been shown to play a significant role in inhibiting growth in a human cervical carcinoma cell line CaSki associated with human papillomavirus (HPV) type 16. EGCg significantly inhibited growth, likely by affecting cell proliferation in the G1 phase and negatively influencing gene expression patterns in CaSki cells, thereby inducing apoptosis. Similar results have been observed in human prostate cancer cells where EGCg affected mitogenesis and induced apoptosis by causing G0/G1 phase cell cycle arrest. Induction of apoptosis in the G1 phase and cell cycle arrest has also been observed in ovarian and liver cancer cell lines. In androgen-sensitive human prostate carcinoma cells, LNCaP, EGCg has been shown to induce apoptosis through stabilization via phosphorylation of critical serine residues on p53 and negative regulation of nuclear factor-κB (NF-κB) activity, a transcription regulatory family. Upregulation of p53 expression has also been seen in liver cancer cells, and a decrease in NF-κB levels and activity was observed in breast cancer cells through inhibition of the Her-2/neu signaling pathway. Additionally, EGCg has been associated with telomerase inhibition, a potential selective target for cancer therapy. However, despite various investigated mechanisms including modulation of signaling pathways, regulation of gene expression, induction of apoptosis, and inhibition of cell proliferation and transformation, the exact mechanism of action of EGCg in inhibiting neoplastic growth remains uncertain.
EGCg also influences immunostimulation and anti-inflammatory responses by suppressing the release of immune inhibitory agents while inducing the release of immune stimulatory agents. The anti-inflammatory activity of EGCg may be associated with an antioxidant function mediated by non-antioxidant mechanisms. This includes the ability of EGCg to modulate signaling pathways and inhibit the activity of transcription factors such as NF-κB and activator protein 1 (AP-1). The antioxidant function of EGCg is characterized by its ability to scavenge reactive oxygen species (ROS), which plays a significant role in enhancing its anti-inflammatory properties. For instance, the downstream effect of inhibiting NF-κB activation results in the downregulation of key neutrophil chemoattractants and inflammatory mediator interleukin-8 (IL-8). Additionally, EGCg modulates inflammatory signaling pathways and negatively impacts the migration of T cells to inflammation sites. Furthermore, catechins activate T lymphocytes and induce the release of tumor necrosis factor-alpha (TNF-α) and interferon-gamma (IFN-γ). The immunostimulatory activity of catechins involves stimulating macrophages to release cytokines, promoting the recruitment of monocytes, dendritic cells, lymphocytes, natural killer cells, and T helper cells.
EGCg has demonstrated a broad spectrum of antimicrobial activity against bacteria and fungi, including growth inhibition of Escherichia coli, Staphylococcus aureus, and Trichophyton sp. Of particular note is the activity of catechins like EGCg against rotavirus, enterovirus, adenovirus, human immunodeficiency virus (HIV), Epstein-Barr virus (EBV), and influenza A and B.
Catechins have exhibited antivirulent activity against HIV through several steps of the HIV life cycle. EGCg not only blocks HIV binding and cell entry but also competitively inhibits HIV-1 DNA polymerase and reverse transcriptase, thereby eliminating viral particles and virus production. Viral transcription is also affected in the presence of EGCg, resulting in a significant decrease in messenger ribonucleic acid (mRNA) expression. Furthermore, it has been shown that EGCg inhibits HIV infection and replication without inducing any apoptosis or necrosis.
By inhibiting the transcription of immediate-early genes, EGCg inhibits the expression of EBV viral protein, thus preventing the initiation of the EBV lytic cycle. In MDCK cells, EGCg affects the infectivity of influenza A and B viruses by agglutinating the virus, blocking the attachment of viral particles to target cell receptors, and preventing the absorption of the virus by MDCK cells. Additionally, EGCg has been shown to inhibit viral replication by preventing the acidification of endosomes and lysosomes, important steps in viral uncoating and replication.
Sinecatechins are produced from the extract of Camellia sinensis green tea leaves containing 15% to 85% catechins, with EGCg representing over 55% of the primary catechin. Approved for prescription use by the United States Food and Drug Administration (FDA), sinecatechins ointment is indicated for the topical treatment of external genital and perianal warts (EGW). Phase 3 clinical trials involving over 1,000 male and female patients with EGW treated with sinecatechins ointment (15%) for up to 16 weeks resulted in statistically superior complete clearance rates of all warts (baseline and new warts) in treated patients compared to controls (54.9% vs. 35.4%, respectively, p <0.001). Additionally, low recurrence rates (6.8%) were demonstrated in all patients where warts were completely cleared, compared to 15% recurrence in previous clinical studies measuring only the clearance of baseline warts, representing a more relevant therapeutic endpoint.

Figure 1
Structure of green tea catechins.
EGCG=epigallocatechin-3-gallate
While the molecular mechanisms underlying the antiviral and anticarcinogenic potentials of green tea catechins have been elucidated in numerous studies recently, the mechanism of action (MOA) of sinecatechins in the eradication of EGW is not yet known. As an anticarcinogenic agent, it has been demonstrated that green tea catechins intervene in various biochemical pathways related to tumor proliferation, mitogenic signal transduction, cell cycle progression, neoplastic cell transformation, and inflammation. As an antiviral agent, catechins have exhibited the ability to inhibit reverse transcriptase and viral transcription, interfere with viral replication, and prevent viral particles from adhering to target cell receptors.
To investigate the potential of sinecatechins to inhibit growth in HPV-positive tumor cell lines, four cervical carcinoma cell lines were treated with sinecatechins. The aim of this study was to explore the potential of catechins to inhibit growth in HPV-infected human cervical carcinoma cell lines. This included examining the effects of catechins on the viability of HPV-16 positive cells (CaSki and SiHa) and HPV-18 positive cells (HeLa and C4-I), evaluating the kinetics of the antiproliferative effects of sinecatechins on cervical carcinoma cell lines, and determining whether the observed efficacy in viability assays (WST-1) stemmed from a delay in cell proliferation or a decrease in total cell count due to cell death.
Methods In the study, three batches of green tea extract were used from Tokyo Food Techno Co. Ltd., Tokyo, Japan. Additionally, green tea extracts from different suppliers were tested for comparison, including Honson Industries Ltd. (Markham, Canada), Wuxi Green Power BioProduct Ltd. (Wuxi, Jiangsu Province, China), Amax NutraSource Inc. (California, USA), and Emil Flachsmann AG (Wädenswil, Switzerland) (see Table 1 for batch numbers and composition). The active ingredient sinecatechins were synthesized from these green tea extracts. A molecular weight of 400 g/mol was assumed for sinecatechins calculations, and they were dissolved in 100 mM concentration in 0.9% NaCl. They were also diluted using an appropriate cell culture medium.
TABLE 1
Composition of green tea extract batches used in experiments.
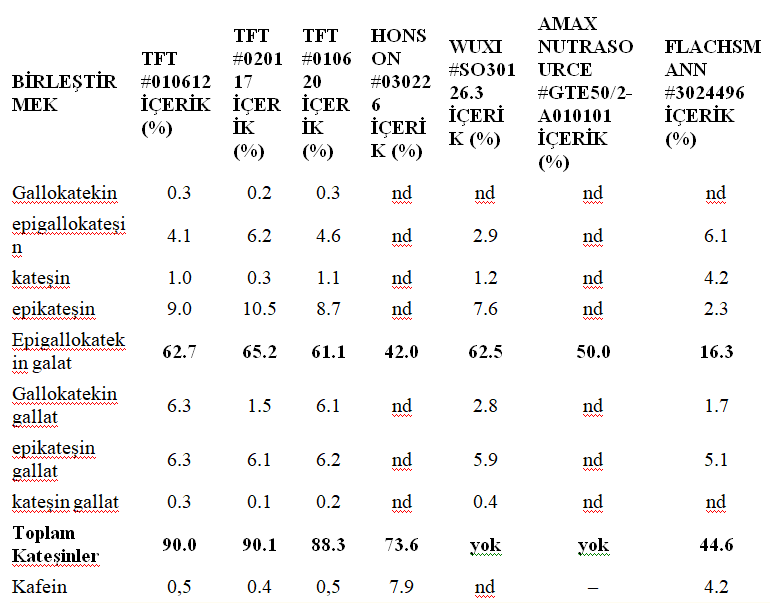
ND=not determined; NA=not applicable
Cell culture. Human cervical carcinoma cell lines CaSki, SiHa (both carrying genomic HPV-16 copies), HeLa, and C4-I (both carrying genomic HPV-18 copies) were obtained from the American Type Culture Collection (Manassas, Virginia, USA). CaSki cells were cultured in RPMI 1640 containing 10 mM HEPES buffer, 2 mM L-glutamine, 1 mM sodium pyruvate, and 10% heat-inactivated fetal calf serum (FCS, Invitrogen, Karlsruhe, Germany). HeLa and SiHa cells were cultured in Dulbecco’s Modified Eagle Medium (DMEM) supplemented with 2 mM L-glutamine, 1 mM sodium pyruvate, and 1% non-essential amino acids (Invitrogen). C4-I cells were cultured in Waymouth MB 752/1 medium supplemented with 10% heat-inactivated FCS (Invitrogen).
Viability assay (WST-1). CaSki, SiHa, HeLa, and C4-I cells were seeded into 96-well flat-bottom plates. Four hours after seeding, the cells were treated with increasing concentrations of sinecatechins. Two days later, a WST-1 assay was performed as per the manufacturer’s instructions (Roche Diagnostics, Mannheim, Germany). This colorimetric viability assay quantifies cell viability and proliferation based on the cleavage of the tetrazolium salt WST-1 by mitochondrial dehydrogenases in viable cells. The cells were incubated with the WST-1 reagent for 4 to 6 hours. Subsequently, the conversion of WST-1 was measured at 450 nm with a reference wavelength of 655 nm in an enzyme-linked immunosorbent assay (ELISA) reader. The amount of converted reagent is proportional to the number of viable cells. Inter-assay variability was approximately 20%, whereas intra-assay variability reached up to 40%. The GI50 value was calculated using a four-parameter sigmoid equation by SigmaPlot 2004 for Windows Version 9.0.
CaSki cells seeded into 96-well flat-bottom plates (4.5×10^3 cells/well) were incubated with varying concentrations of sinecatechins for increasing time periods. The medium was changed for cultures processed for less than 48 hours, meaning the total incubation time for all samples was 48 hours. As a negative control, cells were incubated with medium only, as described above. Viability was analyzed using the WST-1 assay. Cells were incubated with the WST-1 reagent for four hours. Samples were analyzed in triplicate.
For the analysis of different green tea extract suppliers and different groups of sinecatechins, CaSki cells were seeded into 96-well flat-bottom plates at a density of 4.5×10^3 cells/well. Green tea extracts were added, and cells were cultured for 48 hours. Viability was determined using the WST-1 assay as described above.
Clonogenic analysis. This assay was established to determine whether the efficacy observed in the viability assay (WST-1) was due to a delay in cell proliferation or a decrease in total cell count resulting from cell death. Therefore, HeLa and SiHa cells were seeded into six-well plates at low density (0.8×10^2 cells/well) and cultured for three days until small cell colonies developed. Cells were treated with increasing concentrations of sinecatechins (0.2mM–1mM) in medium containing 2.5% FCS for two hours. Subsequently, cells were cultured in medium containing 10% FCS for up to 23 days until countable colonies formed. Medium change was performed regularly.
Colonies were stained with methylene blue (Merck, Darmstadt, Germany) for 10 minutes at room temperature. Plates were then rinsed several times with distilled water and air-dried. Samples were tested in triplicate.
Results
Antiproliferative effect of sinecatechins on cervical carcinoma cells. To analyze whether sinecatechins have an effect on the viability of HPV-16 and HPV-18 positive cells, two HPV-16 positive (CaSki, SiHa) and two HPV-18 positive cell lines (HeLa, C4-I) were tested. All four cervical carcinoma cell lines were incubated with increasing concentrations of sinecatechins (2–2500uM; TFT Lot. #010612) for 48 hours, followed by analysis of cell viability using a WST-1 assay. Growth of all four cell lines was inhibited by sinecatechins in a concentration-dependent manner (Figure 2). Total growth inhibition by sinecatechins was achieved at concentrations of 39 uM for C4-I cells and 625 uM for CaSki, SiHa, and HeLa cells. With a calculated GI50 of approximately 34uM and a maximum inhibitory effect at 39uM, C4-I cells appeared to be the most sensitive to the cytotoxic potential of sinecatechins (Figure 2D). CaSki, SiHa, and HeLa cells appeared to be less sensitive to the cytotoxic potential of sinecatechins compared to C4-I cells, with GI50 values ranging from 160µM to 360µM (Figure 2A–C). Interestingly, the potential of sinecatechins to inhibit growth increased when cells were incubated with medium containing reduced amounts of FCS (2.5%). These data demonstrate the concentration-dependent antiproliferative effect of sinecatechins on HPV-16 and HPV-18 positive cervical carcinoma cell lines.
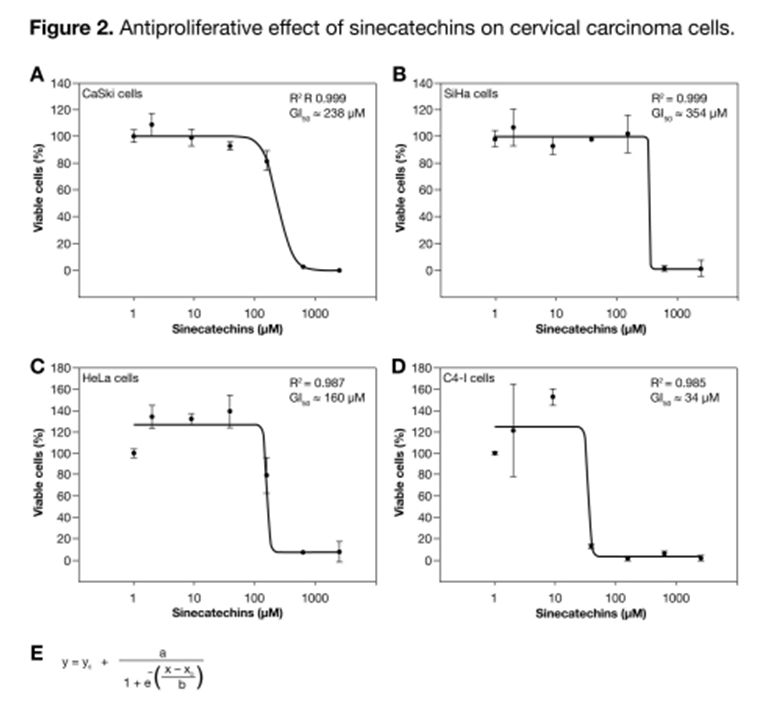
Figure 2
Antiproliferative effect of sinecatechins on cervical carcinoma cells. Cells were seeded into 96-well plates with cell numbers indicated. Cells were incubated with increasing concentrations of sinecatechins (TFT Lot #010612) for 48 hours. Viability was analyzed using the WST-1 assay. Data were evaluated using a sigmoidal four-parameter curve equation (E) with SigmaPlot 2004 for Windows Version 9.0. Results represent the mean ± SD obtained from three replicates and are expressed as the percentage of viable cells compared to untreated cells. One representative of two experiments showing similar decreases in cell viability after treatment with sinecatechins is depicted. TFT=Tokyo Food Techno Co., Ltd.
Effect of incubation duration on the inhibitory effect of sinecatechins on cell growth. CaSki cells were incubated with different concentrations of sinecatechins ranging from 78 uM to 2.5 mM for 1, 2, 4, 20, and 48 hours to analyze the kinetics of the antiproliferative effect on cervical carcinoma cell lines. Medium was changed for cultures processed for less than 48 hours (i.e., total incubation time was 48 hours for all samples). Viability was analyzed using the WST-1 assay. Cells were incubated only with medium as a negative control. In Figure 3, only two concentrations, 313uM and 625uM, are depicted because they were primarily affected by the treatment duration with sinecatechins. A one-hour treatment was sufficient to detect a growth-inhibitory effect at 313uM and 625uM (Figure 3). Kinetic analysis showed that the growth inhibitory potential increased with longer incubation periods and reached a maximum at 48 hours. The same type of experiment was performed using HeLa cells. The result was similar, except that HeLa cells were more sensitive to treatment with sinecatechins and resulted in a 75% inhibition at 313 uM after a four-hour treatment.
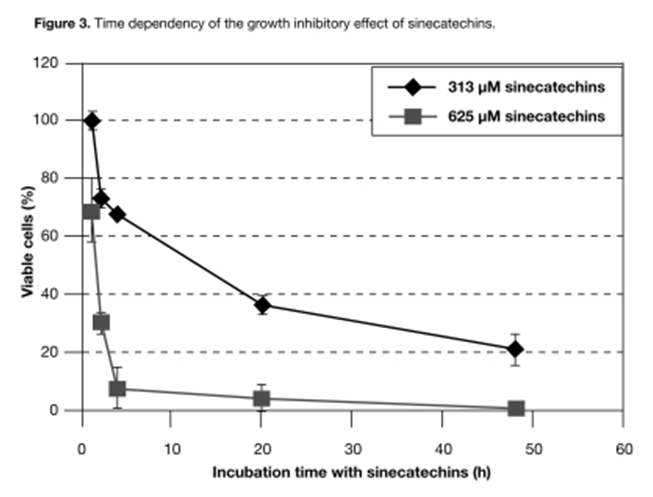
Figure 3
Time-dependency of the growth inhibitory effect of sinecatechins. CaSki cells (4.5×10^3 cells/well) seeded into 96-well plates were incubated with sinecatechins at various concentrations ranging from 78uM to 2.5mM (TFT Lot #010612) for increasing time periods. Only two concentrations, 313μM and 625μM, are depicted as they were primarily affected by the varying treatment duration. Medium was changed for cultures processed for less than 48 hours (i.e., total incubation time was 48 hours for all samples). Cells were incubated only with medium without sinecatechins as a negative control. Viability was analyzed using the WST-1 assay. Results represent the mean ± SD obtained from three replicates and are expressed as the percentage of viable cells compared to untreated cells. TFT=Tokyo Food Techno Co., Ltd.
Effect of green tea extract from different suppliers on cell growth. To investigate whether green tea extracts from different suppliers have different growth inhibitory potentials, three different batches from TFT (Tokyo Food Techno Co. Ltd., Tokyo, Japan) and one batch each from Honson Industries Ltd., Wuxi (Wuxi Green Power BioProduct Ltd.), Amax NutraSource Inc., and Emil Flachsmann AG were used (see Table 1 for composition). CaSki cells were treated with seven different concentrations from each batch ranging from 39uM to 2.5mM. Only one out of seven batches showed a decrease in the potential to inhibit CaSki cell growth (Figure 4). Epigallocatechin gallate (EGCg) is the major component of polyphenols in green tea extract. The content of EGCg varied between 65% and 42% in batches showing similar growth inhibition potential. Only in the sample obtained from Flachsmann (#3024496), there was a significant decrease in EGCg content (16%) and total catechin content (44.6%), and a reduced growth inhibitory effect was also detected in this sample.
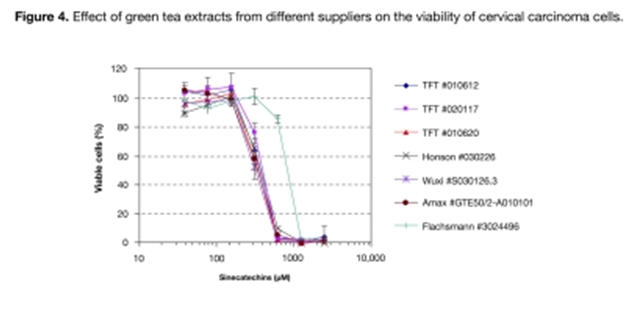
Figure 4
Effect of green tea extracts from different suppliers on the viability of cervical carcinoma cells. CaSki cells were seeded into 96-well plates (4.5×10^3 cells/well) and incubated with sinecatechins at increasing concentrations from different suppliers (TFT Lot #010612, TFT Lot #020117, TFT Lot #010620, Honson Lot #030226, Wuxi Lot # SO30126.3, Amax NutraSource Inc. Batch #GTE50/2-A010101, Flachsmann Batch #3024496) for 48 hours. Viability was analyzed using the WST-1 assay. Results represent the mean ± SD obtained from three replicates and are expressed as the percentage of viable cells compared to untreated cells. TFT=Tokyo Food Techno Co., Ltd.
Effect of sinecatechins on cell growth using clonogenic analysis. A clonogenic analysis was conducted to determine whether the observed effect in viability assays (WST-1) was due to a delay in cell proliferation or a decrease in the total number of cells resulting from cell death. HPV-16 and HPV-18 positive cervical carcinoma cell lines (SiHa and HeLa, respectively) were seeded into six-well plates and treated with increasing concentrations of sinecatechins for two hours. Subsequently, the medium was changed to remove the sinecatechins, and the cells were incubated for up to 17 and 23 days for HeLa and SiHa cells, respectively.
The number of clones decreased with increasing concentrations of sinecatechins in both cell lines (Figure 5). The highest reduction in clone numbers for HeLa cells was observed at the lowest concentration of sinecatechins analyzed, which was 200uM. The clone numbers decreased from approximately 50 to 10 clones. Using higher sinecatechin concentrations (up to 1mM) did not result in a further noticeable decrease in clone numbers. Treatment with sinecatechins in SiHa cells resulted in a more consistent decrease in clone numbers with increasing drug concentrations.
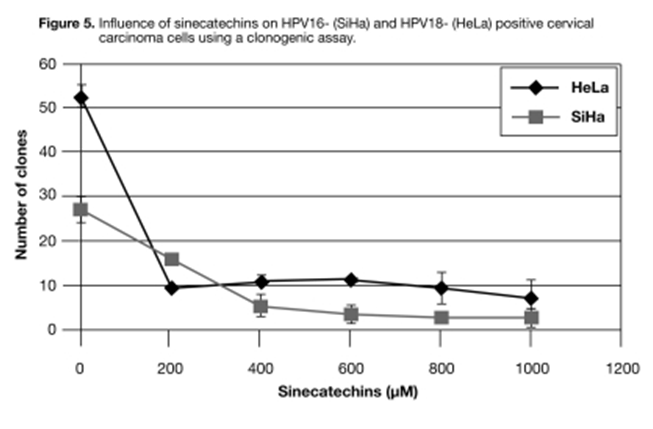
Figure 5
Effect of sinecatechins on HPV16- (SiHa) and HPV18- (HeLa) positive cervical carcinoma cells using clonogenic analysis. Cells seeded in six-well plates (0.8×10^2 cells/well) were allowed to grow until day 3. Subsequently, they were incubated with increasing concentrations of sinecatechins (TFT Lot #020117) for two hours in medium containing 2.5% FCS. The medium was then changed, and the cells were incubated in fresh medium without sinecatechins but containing 10% FCS until day 13 (HeLa) or day 23 (SiHa). Single clones were counted after staining with methylene blue. Results represent the mean ± SD from duplicates (HeLa) or triplicates (SiHa). This experiment was conducted twice with different cell numbers, showing the same reduction in clone numbers after treatment with sinecatechins. TFT=Tokyo Food Techno Co., Ltd. FCS=fetal calf serum.
Almost complete eradication of clones was achieved with 600uM sinecatechins (Figure 5). The experiment was repeated with different cell numbers, and the same effect demonstrating the long-term cytotoxic potential of sinecatechins in cervical cancer cell lines was observed. Thus, sinecatechins not only delay cell proliferation but also induce cell death.
Discussion
Sinecatechins were investigated for their potential to inhibit growth in HPV-infected human cervical carcinoma cell lines, including two HPV-16 positive (CaSki and SiHa) and two HPV-18 positive (HeLa and C4-I) cervical cell lines. Sinecatechins inhibited cell growth by 50% in cervical cancer cell lines CaSki, SiHa, and HeLa at concentrations ranging from 160 to 360µM. Overall, the growth of all four cell lines was consistently inhibited in a concentration-dependent manner by sinecatechins. C4-I cells were the most sensitive to treatment with sinecatechins, showing a GI50 of approximately 34uM and a maximum inhibitory effect at 39uM. Total inhibition was achieved at 625uM for CaSki, SiHa, and HeLa cells, and at 39uM for C4-I cells. Kinetic analysis of growth inhibition showed that the effect increased over time, reaching a maximum at 48 hours. Additionally, green tea extracts with EGCg content ranging from 42% to 65% showed no difference in growth inhibition potential in a 48-hour analysis. Only the Flachsmann batch with 16% EGCg content exhibited significantly reduced growth inhibition. Clonogenic experiments confirmed the inhibitory effect on cell growth and demonstrated that sinecatechins not only inhibited metabolic activity but also induced cell death.
The concentration range of sinecatechins affecting the growth of tumor cell lines in this study is consistent with findings reported by others. Ahn et al. showed that EGCg inhibited the growth of CaSki cells with a GI50 of approximately 35uM in a 1-2 day assay. Yokoyama et al. reported a 50% reduction in cell growth in SiHa cells at 100uM after seven days of treatment (p <0.01) and a 25% inhibition in HeLa cells at 100uM after four days (p <0.05). Morré et al. showed a GI50 of approximately 2uM for inhibition of HeLa growth in a 72-hour assay. Differences in GI50 values may be due to variations in incubation time, cell density, or differences in the assay used to detect growth inhibition.
Interestingly, the study by Morré et al. demonstrated that when individual green tea catechins (EGCg, GCg, ECg, EGC, and EC) were tested, EGCg was the most potent, followed by GCG. This is consistent with the data obtained from the current study, indicating that catechins containing a gallate group are the most effective catechins in reducing cell viability. Morré et al. also reported that the amount of EGCg required to inhibit cell growth was reduced by approximately 10-fold with a combination of inactive catechin EC, showing a synergistic effect between EC and EGCg.
Discussion
The potential of green tea extracts to inhibit growth has been linked to the induction of apoptosis, cell cycle arrest, and telomerase inhibition. Recent articles have suggested that the cytotoxic effects of green tea catechins in in vitro assays are also induced by increased hydrogen peroxide (H2O2) levels in the cell culture environment. It has been demonstrated that ROS production is induced by some polyphenols during an auto-oxidation process under specific conditions in cell culture media. This antioxidant function plays a significant role in enhancing the anti-inflammatory properties of EGCg, involving the activation of transcription factors (NF-κB and AP-1), leading to the downregulation of inflammatory mediators. It is noteworthy to mention that the effect of EGCg on metabolic activity, which accentuates its antiproliferative effect and contributes to its overall cancer-preventive function, could also potentially direct its antiviral activity.
Overall, this study demonstrates the potential of sinecatechins to inhibit the growth of cervical cancer cells, which may partially contribute to their overall cancer-preventive function. The similarity in the growth-inhibitory potentials of various batches of green tea extracts used to synthesize sinecatechin drug substance may imply that commercially available sinecatechins perform similarly. While the exact MOA of sinecatechins is not known, their known antioxidant, immunostimulatory, and antiproliferative activities, in addition to their antiviral effects, may contribute to the high efficacy rates and low recurrence rates observed after topical application of EGW treatment. Besides antioxidant activity, the FDA has not approved the presence of any additional activity on product labeling. Most EGW treatments applied by providers and by patients rely on destructive methods to eradicate lesions, apart from imiquimod, which has immunostimulatory activity. Head-to-head studies with other topical EGW therapies administered by patients (e.g., imiquimod, podophyllotoxin) have not been conducted; however, EGW clearance rates reported in the published literature for other treatments are lower than those observed after treatment with sinecatechins. More importantly, higher recurrence rates have been reported compared to sinecatechins ointment (13-19% for imiquimod, up to 40% for cryotherapy, and up to 91% for podophyllotoxin), with 15%.
Solution
The inhibitory effects of green tea on experimental carcinogenesis and viral replication and inhibition have been demonstrated in various studies, either in vitro or in vivo. Described as antineoplastic agents, catechins are reported to inhibit cell growth and induce apoptosis in several human carcinoma cells. However, as antiviral agents, catechins have been shown to inhibit viral replication through disruption at various stages of the viral life cycle and to prevent infectivity through processes like hemagglutination. Unlike anticarcinogenic processes, the antiviral ability of catechins has not been attributed to apoptosis or necrosis. The activity of sinecatechins demonstrated in this study provides additional insights into its mechanism of action, particularly concerning EGW clearance.
Acknowledgments
The author gratefully acknowledges the funding and execution of the study presented in this manuscript by MediGene AG, Planegg/Martinsried, Germany, and their employees. The author also acknowledges the writing and editorial assistance provided by Priya Karkhanis and Malik Cobb from PA-C.
Footnotes
DISCLOSURE: Dr. Tyring has conducted clinical trials initiated by the investigator and sponsored by PharmaDerm®, a division of Fougera Pharmaceuticals Inc.
REFERENCES
1. Cooper R, Morré DJ, Morré DM. Yeşil çayın tıbbi faydaları: Bölüm I Kanser dışı sağlık yararlarının gözden geçirilmesi. J Alternatif Tamamlayıcı Med. 2005; 11 (3):521–528. [ PubMed ] [ Google Akademik ]
2. Meltzer SM, Monk BJ, Tewari KS. Dış genital siğillerin tedavisi için yeşil çay kateşinleri. Ben J Obstet Gynecol. 2009; 200 (3):233.e1–233.e7. [ PubMed ] [ Google Akademik ]
3. Balentine DA, Wiseman SA, Bouwens LC. Çay flavonoidlerinin kimyası. Crit Rev Food Sci Nutr. 1997; 37 (8):693–704. [ PubMed ] [ Google Akademik ]
4. Ahn WS, Huh SW, Bae SM ve ark. Yeşil çayın önemli bir bileşeni olan EGCg, apoptoz, G1 tutuklaması ve gen ekspresyonunun düzenlenmesi yoluyla bir insan servikal hücre dizisi olan CaSki hücrelerinin büyümesini engeller. DNA Hücre Biol. 2003; 22 (3):217–224. [ PubMed ] [ Google Akademik ]
5. Rahman I, Biswas SK, Kirkham PA. Diyet polifenolleri ile iltihaplanma ve redoks sinyalinin düzenlenmesi. Biochem Eczacılık. 2006; 72 (11):1439–1452. [ PubMed ] [ Google Akademik ]
6. Masuda M, Suzui M, Weinstein IB. Epigallocatechin-3-gallat’ın insan baş ve boyun skuamöz hücreli karsinom hücre hatlarında büyüme, epidermal büyüme faktörü reseptörü sinyal yolları, gen ekspresyonu ve kemosensitivite üzerindeki etkileri. Klinik Kanser Arş. 2001; 7 (12):4220–4229. [ PubMed ] [ Google Akademik ]
7. Huh SW, Bae SM, Kim YW ve diğerleri. (-)-Epigallocatechin-3-gallat’ın yumurtalık karsinomu hücre dizileri üzerindeki antikanser etkileri. Jinekol Onkol. 2004; 94 (3):760–768. [ PubMed ] [ Google Akademik ]
8. Kuo PL. Lin CC. Yeşil çay bileşeni (-)-epigallocatechin-3-gallate, Hep G2 hücre proliferasyonunu inhibe eder ve p53’e bağlı ve Fas aracılı yollar yoluyla apoptozu indükler. J Biomed Sci. 2003; 10 (2):219–227. [ PubMed ] [ Google Akademik ]
9. Gupta S, Ahmad N, Nieminen AL, Mukhtar H. Androjene duyarlı ve androjene duyarlı olmayan insan prostat karsinomunda yeşil çay bileşeni (-)-epigallocatechin-3-gallate tarafından büyüme inhibisyonu, hücre döngüsü düzensizliği ve apoptozun indüksiyonu hücreler. Toksikol Uygulaması Pharmacol. 2000; 164 (1):82–90. [ PubMed ] [ Google Akademik ]
10. Hastak K, Gupta S, Ahmad N, et al. LNCaP hücrelerinin epigallocatechin-3-gallate ile indüklenen apoptozunda p53 ve NF-κB’nin rolü. onkojen. 2003; 22 :4851–4859. [ PubMed ] [ Google Akademik ]
11. Pianetti S, Guo S, Kavanagh KT, Sonenshein GE. Yeşil çay polifenol epigallocatechin-3 gallate, meme kanseri hücrelerinin Her-2/neu sinyalini, proliferasyonunu ve dönüştürülmüş fenotipini inhibe eder. Kanser Arş. 2002; 62 (3):652–655. [ PubMed ] [ Google Akademik ]
12. Yokoyama M, Noguchi M, Nakao Y, et al. Çay polifenol, (-)-epigallocatechin gallate, servikal hücre hatlarında büyüme, apoptoz ve telomeraz aktivitesi üzerindeki etkileri. Jinekol Onkol. 2004; 92 (1):197–204. [ PubMed ] [ Google Akademik ]
13. Yang CS, Yang GY, Landau JM ve ark. Çay ve çay polifenolleri, hücre hiperproliferasyonunu, akciğer tümör oluşumunu ve tümör ilerlemesini engeller. Exp Akciğer Res. 1998; 24 (4):629–639. [ PubMed ] [ Google Akademik ]
14. Chang LK, Wei TT, Chiu YF ve ark. (-)-epigallocatechin gallate tarafından Epstein-Barr virüsü litik döngüsünün inhibisyonu. Biochem Biophys Res Kom. 2003; 301 (4):1062–1068. [ PubMed ] [ Google Akademik ]
15. Toda M, Okubo S, Ikigai H, et al. Çay kateşinlerinin Vibrio cholerae O1 tarafından deneysel enfeksiyona karşı koruyucu aktivitesi. Mikrobiyoloji İmmunol. 1992; 36 (9):999–1001. [ PubMed ] [ Google Akademik ]
16. Weber JM, Ruzindana-Umunyana A, Imbeault L, Sircar S. Yeşil çay kateşinleri tarafından adenovirüs enfeksiyonu ve adenainin inhibisyonu. Antivir Res. 2003; 58 (2):167–173. [ PubMed ] [ Google Akademik ]
17. Yamaguchi K, Honda M, Ikigai H, et al. (-)-Epigallocatechin gallate’in insan immün yetmezlik virüsü tip 1 (HIV-1) Antivir Res. 2002; 53 (1):19–34. [ PubMed ] [ Google Akademik ]
18. Nakane H, Ono K. Bazı kateşin türevlerinin, bazı kateşin türevleri tarafından insan immün yetmezlik virüsü ters transkriptaz ve hücresel deoksiribonükleik ve ribonükleik asit polimerazlar ve çeşitli DNA ve RNA polimerazların aktiviteleri üzerindeki farklı önleyici etkileri. Biyokimya. 1990; 29 :2841–2845. [ PubMed ] [ Google Akademik ]
19. Fassina G, Buffa A, Benelli R, et al. Aday bir anti-HIV ajanı olarak yeşil çaydan elde edilen polifenolik antioksidan (-)-epigallocatechin-3-gallate. AIDS. 2002; 16 (6):939–941. [ PubMed ] [ Google Akademik ]
20. Nakayama M, Suzuki K, Toda M, et al. İnfluenza virüsünün enfektivitesinin çay polifenolleri tarafından inhibisyonu. Antivir Res. 1993; 21 (4):289–299. [ PubMed ] [ Google Akademik ]
21. Song JM, Lee KH, Seong BL. Yeşil çaydaki kateşinlerin grip virüsü üzerindeki antiviral etkisi. Antiviral Çöz. 2005; 68 (2):66–74. [ PubMed ] [ Google Akademik ]
22. Imanishi N, Tuji Y, Katada Y, et al. MDCK hücrelerinde influenza A ve B virüslerinin büyümesi üzerinde çay ekstraktının ilave inhibitör etkisi. Mikrobiyoloji İmmunol. 2002; 46 (7):491–494. [ PubMed ] [ Google Akademik ]
23. Okubo S, Toda M, Hara Y, Shimamura T. Trichophyton. Fujieda, Japonya: Mitsui Norin Co., Gıda Araştırma Laboratuvarları; Çay ekstresi ve kateşinlerin antifungal ve fungisidal aktivitelerine karşı. [ PubMed ] [ Google Akademik ]
24. Mukoyama A, Ushijima H, Nishimura S, et al. Çay özleri ile rotavirüs ve enterovirüs enfeksiyonlarının inhibisyonu. Jpn J Med Sci Biol. 1991; 44 :181–186. [ PubMed ] [ Google Akademik ]
25. Yang CS, Maliakal P, Meng X. Çay ile karsinojenezin engellenmesi. Annu Rev Pharmacol Toksikol. 2002; 42 :25–54. [ PubMed ] [ Google Akademik ]
26. Veregen Ürün Monografisi. Melville, NY: PharmaDerm, Nycomed US Inc.’in Bir Bölümü; 2010.
27. Tatti S, Stockfleth E, Beutner KR, et al. Polyphenon E ® : dış anogenital siğiller için yeni bir tedavi. Br J Dermatol. 2010; 162 :176–184. [ PubMed ] [ Google Akademik ]
28. Morré DJ, Morré DM, Sun H, et al. Çay kateşin, kanser hücresi proliferasyonunun ve kansere özgü bir hücre yüzey oksidazının (ECTO-NOX) Pharmacol Toxicol’ün inhibisyonunda sinerji oluşturur . 2003; 92 (5):234–241. [ PubMed ] [ Google Akademik ]